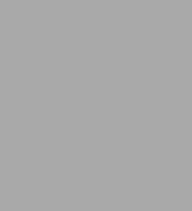
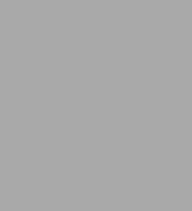
Overview
Product Details
ISBN-13: | 9781119390534 |
---|---|
Publisher: | Wiley |
Publication date: | 09/08/2017 |
Sold by: | JOHN WILEY & SONS |
Format: | eBook |
Pages: | 1216 |
File size: | 28 MB |
Note: | This product may take a few minutes to download. |
About the Author
ÁRPÁD MOLNÁR, DSc, is an Emeritus Professor at the University of Szeged, Hungary, and a Senior Fellow of the Loker Hydrocarbon Research Institute at the University of Southern California.
G. K. SURYA PRAKASH, PhD, is the George A. and Judith A. Olah Nobel Laureate Chair Professor and the Director of the Loker Hydrocarbon Research Institute at the University of Southern California.
Read an Excerpt
Hydrocarbon Chemistry
By George A. Olah Árpad Molnar
John Wiley & Sons
ISBN: 0-471-41782-3Chapter One
GENERAL ASPECTS1.1. HYDROCARBONS AND THEIR CLASSES
Hydrocarbons, as their name indicates, are compounds of carbon and hydrogen. As such, they represent one of the most significant classes of organic compounds (i.e., of carbon compounds). In methane (C[H.sub.4]) the simplest saturated alkane, a single-carbon atom, is bonded to four hydrogen atoms. In the higher homologs of methane (of the general formula [C.sub.n][H.sub.2n+2]) all atoms are bound to each other by single [(sigma ([sigma]), two-electron two-center] bonds with carbon displaying its tendency to form C-C bonds. Whereas in C[H.sub.4] the H : C ratio is 4, in [C.sub.2][H.sub.6] (ethane) it is decreased to 3; in [C.sub.3][H.sub.8] (propane), to 2.67; and so on. Alkanes can be straight-chain (with each carbon attached to not more than two other carbon atoms) or branched (in which at least one of the carbons is attached to either three or four other carbon atoms). Carbon atoms can be aligned in open chains (acyclic hydro-carbons) or can form rings (cyclic hydrocarbons).
Cycloalkanes are cyclic saturated hydrocarbons containing a single ring. Bridged cycloalkanes contain one (or more) pair(s) of carbon atoms common to two (or more) rings. In bicycloalkanes there are two carbon atoms common to both rings. In tricycloalkanes there are four carbon atoms common to three rings suchas in adamantane (tricyclo[3.3.1] decane), giving a caged hydrocarbon structure.
Carbon can also form multiple bonds with other carbon atoms. This results in unsaturated hydrocarbons such as olefins (alkenes, [C.sub.n][H.sub.2n]), specifically, hydrocarbons containing a carbon-carbon double bond or acetylenes (alkynes, [C.sub.n][H.sub.n-2]) containing a carbon-carbon triple bond. Dienes and polyenes contain two or more unsaturated bonds.
Aromatic hydrocarbons (arenes), a class of hydrocarbons of which benzene is parent, consist of cyclic arrangement of formally unsaturated carbons, which, however, give a stabilized (in contrast to their hypothetical cyclopolyenes) delocalized [pi] system.
The H : C ratio in hydrocarbons is indicative of the hydrogen deficiency of the system. As mentioned, the highest theoretical H : C ratio possible for hydrocarbons is 4 (in C[H.sub.4]), although in carbocationic compounds (the positive ions of carbon compound) such as C[H.sup.+.sub.5] and even C[H.sup.2+.sub.6] the ratio is further increased (to 5 and 6, respectively). On the other end of the scale in extreme cases, such as the dihydro or methylene derivatives of recently (at the time of writing) discovered [C.sub.60] and [C.sub.70] fullerenes, the H : C ratio can be as low as ~ 0.03!
An index of unsaturation (hydrogen deficiency) i can be used in hydrocarbons whose value indicates the number of ring and/or double bonds (a triple bond is counted as two double bonds) present (C and H = the number of carbon and hydrogen atoms), i = 0 for methane, for ethene i = 1 (one double bond), for acetylene (ethyne) i = 2, and so on:
i = (2C + 2) - H / 2
The International Union of Pure and Applied Chemistry (IUPAC) established rules to name hydrocarbons. Frequently, however, trivial names are also used and will continue to be used. It is not considered necessary to elaborate here on the question of nomenclature. Systematic naming is mostly followed. Trivial (common) namings are, however, also well extended. Olefins or aromatics clearly are very much part of our everyday usage, although their IUPAC names are alkenes and arenes, respectively. Straight-chain saturated hydrocarbons are frequently referred to as n-alkanes (normal) in contrast to their branched analogs (isoalkanes, i-alkanes). Similarly straight-chain alkenes are frequently called n-alkenes as contrasted with branch isoalkenes (or olefins). What needs to be pointed out, however, is that one should not mix the systematic IUPAC and the still prevalent trivial (or common) namings. For example, ([C[H.sub.3]).sub.2]C=C[H.sub.2] can be called isobutylene or 2-methyl-propene. It, however, should not be called isobutene as only the common name butylene should be affixed by iso. On the other hand, isobutane is the proper common name for 2-methylpropane [[(C[H.sub.3]).sub.3]CH]. Consequently we discuss isobutane-isobutylene alkylation for production of isooctane: high-octane gasoline (but it should not be called isobutane-isobutene alkylation).
1.2. ENERGY-HYDROCARBON RELATIONSHIP
Every facet of human life is affected by our need for energy. The sun is the central energy source of our solar system. The difficulty lies in converting solar energy into other energy sources and also to store them for future use. Photovoltaic devices and other means to utilize solar energy are intensively studied and developed, but at the level of our energy demands, Earth-based major installations by present-day technology are not feasible. The size of collecting devices would necessitate utilization of large areas of the Earth. Atmospheric conditions in most of the industrialized world are unsuitable to provide a constant solar energy supply. Perhaps a space-based collecting system beaming energy back to Earth can be established at some time in the future, but except for small-scale installation, solar energy is of limited significance for the foreseeable future. Unfortunately, the same must be said about wind, ocean waves, and other unconventional energy sources.
Our major energy sources are fossil fuels (i.e., oil, gas, and coal), as well as atomic energy. Fossil energy sources are, however, nonrenewable (at least on our timescale), and their burning causes serious environmental problems. Increased carbon dioxide levels are considered to contribute to the "greenhouse" effect. The major limitation, however, is the limited nature of our fossil fuel resources (see Section 1.5). The most realistic estimates put our overall worldwide fossil resources as lasting for not more than 200 or 300 years, of which oil and gas would last less than a century. In human history this is a short period, and we will need to find new solutions. The United States relies overwhelmingly on fossil energy sources, with only 8% coming from atomic energy and 4% from hydro energy (Table 1.1).
Other industrialized countries utilize to a much higher degree of nuclear and hydroenergy (Table 1.2). Since 1980, concerns about safety and fission byproduct disposal difficulties, however, dramatically limited the growth of the otherwise clean atomic energy industry.
A way to extend the lifetime of our fossil fuel energy reserves is to raise the efficiency of thermal power generation. Progress has been made in this respect, but the heat efficiency even in the most modern power plants is limited. Heat efficiency increased substantially from 19% in 1951 to 38% in 1970, but for many years since then 39% appeared to be the limit. Combined-cycle thermal power generation-a combination of gas turbines-was allowed in Japan to further increase heat efficiency from 35-39% to as high as 43%. Conservation efforts can also greatly contribute to moderate worldwide growth of energy consumption, but the rapidly growing population of our planet (5.4 billion today, but should reach 7-8 billion by 2010) will put enormous pressure on our future needs.
Estimates of the world energy consumption until 2020 are shown graphically in Figure 1.1 in relationship to data dating back to 1960. A rise in global energy consumption of 50-75% for the year 2020 is expected compared with that for 1988. Even in a very limited growth economic scenario the global energy demand is estimated to reach 12 billion tons of oil equivalent (t/oe) by the year 2020.
Our long-range energy future clearly must be safe nuclear energy, which should increasingly free still remaining fossil fuels as sources for convenient transportation fuels and as raw materials for synthesis of plastics, chemicals, and other substances. Eventually, however, in the not too distant future we will need to make synthetic hydrocarbons on a large scale.
1.3. HYDROCARBON SOURCES AND SEPARATION
All fossil fuels (coal, oil, gas) are basically hydrocarbons, deviating, however, significantly in their H : C ratio (Table 1.3).
1.3.1. Natural Gas
Natural gas, depending on its source, contains-besides methane as the main hydrocarbon compound (present usually in concentrations >80-90%)-some of the higher homologous alkanes (ethane, propane, butane). In "wet" gases the amount of [C.sub.2]-[C.sub.6] alkanes is more significant (gas liquids). Typical composition of natural gas of various origin is shown in Table 1.4.
Natural-gas liquids are generally of thermal value only but can be used for dehydrogenation to alkenes. Their direct upgrading to gasoline-range hydrocarbons is also pursued. Natural gas as we know it is of biological origin (not unlike petroleum oil). Large gas reservoirs were discovered and utilized in the twentieth century. Increasingly deeper wells are drilled and deposits under the seas are explored and tapped. An interesting but as yet unproved theory by Gold holds that hydrocarbons may also be formed by slow outgassing of methane from vast deep deposits dating back to the origin of our planet. Besides biologically derived oil and gas, "deep" carbon compounds trapped in the Earth's crust are subject to intense heat, causing them to release hydrocarbons that migrate toward the Earth's surface, where they are trapped in different stratas. Seepage observed at the bottom of the oceans and finds of oil during drilling into formations (such as granite) where no "biogenic" oil was expected are cited as proof for "abiogenic" hydrocarbons. If abiogenic methane and other hydrocarbons exist (although most geologists presently disagree), vast new reserves would become available when improved drilling technology is developed to reach deeper into the Earth's crust.
Other vast yet untapped reserves of natural gas (methane) are locked up as hydrates under the permafrost in Siberia. Methane gas hydrates are inclusion compounds of C[H.sub.4]*n]H.sub.2]O composition. Their amount is estimated to equal or exceed known conventional natural-gas reserves. Their economical utilization, however, remains a challenge. Significant amounts [[less than or equal to] 500 million tons per year (Mt/y)] of natural methane is also released into the atmosphere from varied sources ranging from marsh lands to landfills, to farm animals. Methane in the atmosphere represents only a small component, although its increase can cause a significant greenhouse effect.
1.3.2. Petroleum or Crude Oil
Petroleum or crude oil is a complex mixture of many hydrocarbons. It is characterized by the virtual absence of unsaturated hydrocarbons consisting mainly of saturated, predominantly straight-chain alkanes, small amounts of slightly branched alkanes, cycloalkanes, and aromatics. Petroleum is generally believed to be derived from organic matter deposited in the sediments and sedimentary rocks on the floor of marine basins. The identification of biological markers such as petroporphyrins provides convincing evidence for the biological origin of oil (see, however, the abovementioned possibility for abiogenic "deep" hydrocarbons). The effect of time, temperature, and pressure in the geological transformation of the organics to petroleum is not yet clear. However, considering the low level of oxidized hydrocarbons and the presence of porphyrins, it can be surmised that the organics were acted on by anaerobic microorganisms and that temperatures were moderate, <200ºC. By comparing the elemental composition of typical crude oils with typical bituminous coals, it is clear why crude oil is a much more suitable fuel source in terms of its higher H : C atomic ratio, generally lower sulfur and nitrogen contents, very low ash content, (probably mostly attributable to some suspended mineral matter and vanadium and nickel associated with porphyrins), and essentially no water content.
Finally, it is interesting to mention that the most recent evidence shows that even extraterrestrially formed hydrocarbons can reach the Earth. The Earth continues to receive some 40,000 tons of interplanetary dust every year. Mass-spectrometric analysis revealed the presence of hydrocarbons attached to these dust particles, including polycyclic aromatics such as phenanthrene, chrysene, pyrene, benzopyrene, and pentacene of extraterrestrial origin (indicated by anomalous isotopic ratios).
Petroleum-a natural mineral oil-was referred to as early as in the Old Testament. The word petroleum means "rock oil" [from the Greek petros (rock) and elaion (oil)]. It had been found over the centuries seeping out of the ground, for example, in the Los Angeles basin (practically next door to where this review is written) and what are now the La Brea Tar Pits. Vast deposits were found in varied places ranging from Europe, to Asia, to the Americas, and to Africa. In the United States the first commercial petroleum deposit was discovered in 1859 near Titus-ville in western Pennsylvania when Edwin Drake and Billy Smith struck oil in their first shallow (~20-m-deep) well. The well yielded 400 gallons (gal) of oil a day (about 10 barrels). The area was known before to contain petroleum that residents skimmed from a local creek's surface, which was thus called "oil creek." The oil-producing first well opened up a whole new industry. The discovery was not unexpected, but provided evidence for oil deposits in the ground that could be reached by drilling into them. Oil was used for many purposes, such as in lamp illumination and even for medical remedies. The newly discovered Pennsylvania petroleum was soon also marketed to degrease wool, prepare paints, fuel steam engines, and power light railroad cars and for many other uses. It was recognized that the well oil was highly impure and had to be refined to separate different fractions for varied uses (see Section 1.4). The first petroleum refinery, a small stilling operation, was established in Titusville in 1860. Petroleum refining was much cheaper than producing coal oil (kerosene), and soon petroleum became the predominant source for kerosene as an illuminant. In the 1910s the popularity of automobiles spurred the production of gasoline as the major petroleum product. California, Texas, Oklahoma, and more recently Alaska provided large petroleum deposits in the United States, whereas areas of the Middle East, Asia, Russia, Africa, South America, and more recently of the North Sea became major world oil production centers.
The daily consumption of crude oil in the United States is about 16-17 million barrels.
Continues...
Excerpted from Hydrocarbon Chemistry by George A. Olah Árpad Molnar Excerpted by permission.
All rights reserved. No part of this excerpt may be reproduced or reprinted without permission in writing from the publisher.
Excerpts are provided by Dial-A-Book Inc. solely for the personal use of visitors to this web site.
Table of Contents
Volume 1Preface to the Third Edition xiii
Preface to the Second Edition xv
Preface to the First Edition xvii
Introduction xix
Introduction and General Aspects 1
1.1 Hydrocarbons and Their Classes 1
1.2 Energy–Hydrocarbon Relationships 2
1.3 Hydrocarbon Sources 4
Extraterrestrial Hydrocarbons 15
1.4 Hydrocarbon Production from Natural Sources 16
1.5 Hydrocarbon Synthesis 20
1.6 Nonrenewable and Renewable Hydrocarbons 27
1.7 Regenerative Hydrocarbons from CO2 Emission Capture and Recycling 29
1.8 Hydrocarbon Functionalization Reactions 30
1.9 Use of Hydrocarbons, Petroleum Oil 35
1.9.1 Energy Generation, Storage, and Delivery: Heating 36
1.9.2 Transportation Fuels 36
1.9.3 Chemical Products, Plastics, and Pharmaceuticals 38
References 38
Hydrocarbons from Petroleum and Natural Gas 49
2.1 Cracking 49
2.2 Reforming 62
Hydroforming 64
Metal-Catalyzed Reforming 65
2.3 Dehydrogenation with Olefin Production 71
Heterogeneous Catalysts 73
Homogeneous Catalysts 78
C2–C3 Alkenes 85
C4 Alkenes 86
Buta-1,3-diene and Isoprene 87
Higher Olefins 88
Styrene 88
2.4 Upgrading of Natural-Gas Liquids 89
2.5 Aromatics Production 89
References 102
Synthesis from C Sources 125
3.1 Aspects of C1 Chemistry 126
3.2 Chemical Reduction to Methanol and Oxygenates; Recycling of CO2 127
Heterogeneous Hydrogenation 129
Homogeneous Hydrogenation 137
Ionic Reduction 143
Electrochemical and Electrocatalytic Reduction 143
Photoreduction 146
Enzymatic Reduction 148
3.3 Fischer–Tropsch Chemistry 149
3.4 Oxygenation of Methane 166
Methanol Synthesis 166
3.5 Oligocondensation of Methane 173
3.6 Hydrocarbons from Methane Derivatives 186
Methanol Conversion to Hydrocarbons 186
Methanol to Hydrocarbon Technologies 196
Methanol to Gasoline 196
Methanol to Olefin 197
Methanol to Propylene 198
References 200
Isomerization 237
4.1 Acid-Catalyzed and Bifunctional Isomerization 238
Mechanism 243
Side-Chain Isomerization 250
Positional Isomerization 250
4.2 Base-Catalyzed Isomerization 262
4.2.1 Alkenes 262
4.3 Metal-Catalyzed Isomerization 266
4.4 Pericyclic Rearrangements 277
4.5 Practical Applications 284
Alkanes 284
Alkenes 285
4.5.2 Isomerization of Xylenes 286
References 287
Alkylations 305
5.1 Acid-Catalyzed Alkylation 305
Alkylolysis (Alkylative Cleavage) 317
Alkylation of Alkenes with Organic Halides 318
Alkylation of Alkynes 320
Alkylation with Carbonyl Compounds: The Prins Reaction 320
Catalysts 324
Alkylation with Alkyl Halides 326
Alkylation with Alkenes 331
Alkylation with Alkanes 335
Alkylation with Other Reagents 338
5.2 Base-Catalyzed Alkylation 350
5.3 Alkylation through Organometallics 352
5.4 Miscellaneous Alkylations 356
5.5 Practical Applications 360
References 369
Addition Reactions 389
6.1 Hydration 389
Production of Alcohols by Hydration of Alkenes 395
Production of Octane-Enhancing Oxygenates 396
Acetaldehyde 397
6.2 HX Addition 398
Alkenes 398
Dienes 403
Alkynes 404
Ethyl Chloride 411
Hydrochlorination of Buta-1,3-diene 411
Vinyl Chloride 411
Ethylene Chlorohydrin 412
Propylene Chlorohydrin 412
Adiponitrile 412
Acrylonitrile 413
6.3 Halogen Addition 413
Vinyl Chloride 422
Chlorination of Buta-1,3-diene 424
6.4 Addition to Form C–N Bonds 424
6.5 Addition to Form C–O, C–S, and C–P Bonds 433
6.6 Hydrometalation 439
Alkenes 440
Dienes 446
Alkynes 448
Alkenes 452
Dienes 456
Alkynes 457
6.7 Halometalation 462
6.8 Solvometalation 465
6.9 Carbometalation 466
6.10 Cycloaddition 471
References 477
Carbonylation and Carboxylation 509
7.1 Carbonylation 509
Hydroformylation in Biphasic Solvent Systems 515
The Use of Heterogeneous Catalysts 516
Hydroformylation of Higher Alkenes 518
Hydroformylation of Internal Alkenes 519
Asymmetric Hydroformylation 520
7.2 Carboxylation 533
Saturated Hydrocarbons 534
Aromatic Hydrocarbons 536
Hydrocarboxylation and hydroesterification 539
Aminocarboxylation 545
Neocarboxylic Acids 547
Hydrocarboxymethylation of Long-Chain Alkenes 547
Propionic Acid 547
Acrylic Acid and Acrylates 548
References 548
Acylation 569
8.1 Acylation of Aromatics 569
New Soluble Catalysts 573
Solid Catalysts 575
The Gattermann–Koch Reaction 577
The Gattermann Reaction 579
Other Formylations 580
8.2 Acylation of Aliphatic Compounds 581
References 586
Index 000
Volume 2
Preface to the Third Edition xi
Preface to the Second Edition xiii
Preface to the First Edition xv
Introduction xvii
Oxidation–Oxygenation 593
9.1 Oxidation of Alkanes 594
Autoxidation of Alkanes 594
Oxidation of Methane 596
Oxidation with Stoichiometric Oxidants 606
Oxidation Catalyzed by Enzymes and Metalloporphyrins 613
Metal-Catalyzed Oxidation in the Homogeneous Phase 616
Oxidation Induced by Heterogeneous Catalysts 619
Metal Oxidants 623
Electrophilic Reagents 624
Oxygenolysis 628
9.2 Oxidation of Alkenes 630
Direct Oxidation with Stoichiometric Oxidants 630
Metal-Catalyzed Epoxidation 635
Epoxidation Catalyzed by Metalloporphyrins 644
Asymmetric Epoxidation 647
Autoxidation 650
Reactions with Singlet Oxygen 650
Bis-Hydroxylation 656
Bis-Acetoxylation 663
Oxidation with Palladium in the Homogeneous Phase 664
Oxidation with Other Reagents 669
Vinylic Acetoxylation 671
Ozonation 673
Mechanism 673
Synthetic Applications 676
Other Oxidants 678
Allylic Hydroxylation and Acyloxylation 681
Oxidation to α,β-Unsaturated Carbonyl Compounds 686
9.3 Oxidation of Alkynes 690
9.4 Oxidation of Aromatics 693
Oxidation to Phenols 693
Ring Acyloxylation 701
Oxidation to Quinones 702
Oxidation to Arene Oxides and Arene Diols 703
Oxidation with Singlet Oxygen 704
Oxidation of Methyl-Substituted Aromatics 706
Oxidation of Other Arenes 708
Benzylic Acetoxylation 711
9.5 Practical Applications 712
Acetic Acid 712
Maleic Anhydride 713
Oxidation of Cyclohexane 715
Oxidation of Cyclododecane 715
sec-Alcohols 715
Ethylene Oxide 716
Propylene Oxide 718
Acetaldehyde and Acetone 719
Vinyl Acetate 719
1,4-Diacetoxybut-2-ene 720
Acrolein and Acrylic Acid 720
Methacrolein and Methacrylic Acid 721
Acrylonitrile 721
Other Processes 722
Phenol and Acetone 722
Benzoic Acid 723
Terephthalic Acid 723
Maleic Anhydride 724
Phthalic Anhydride 725
Anthraquinone 727
References 727
Heterosubstitution 795
10.1 Electrophilic (Acid-Catalyzed) Substitution 795
Halogenation 796
Nitration 798
Sulfuration 799
Halogenation 800
Nitration 804
Sulfonation 808
Synthesis of Sulfoxides and Sulfones 810
Chlorobenzene 811
Nitration of Benzene and Toluene 811
Sulfonation of Benzene and Alkylbenzenes 811
10.2 Free-Radical Substitution 812
Chlorination 812
Fluorination 817
Bromination 818
Iodination 819
Side-Chain Halogenation of Arylalkanes 819
Chlorination of Alkanes 824
Side-Chain Chlorination of Toluene 826
Unsaturated Chlorides 826
Sulfochlorination of Alkanes 827
Nitroalkanes 827
10.3 Formation of C–N Bonds 827
10.4 Formation of Carbon–Metal Bonds 831
Borylation 837
Silylation 840
Al, Ge, and Sn Derivatives 841
10.5 Miscellaneous Derivatives 842
References 843
Reduction–Hydrogenation 863
11.1 Heterogeneous Catalytic Hydrogenation 864
Mechanism 866
Stereochemistry 870
11.2 Homogeneous Catalytic Hydrogenation 886
Mechanism 891
Selectivity and Stereochemistry 893
Asymmetric Hydrogenation 896
11.3 Transfer Hydrogenation 904
11.4 Chemical and Electrochemical Reduction 906
Mechanism 911
Selectivity 911
11.5 Ionic Hydrogenation 913
11.6 Hydrogenolysis of Saturated Hydrocarbons 918
11.7 Practical Applications 931
C2 Hydrorefining 931
C3 Hydrorefining 931
C4 Hydrorefining 931
Gasoline Hydrorefining 932
References 934
Metathesis 959
12.1 Metathesis of Acyclic Alkenes 960
12.2 Alkane Metathesis 973
12.3 Metathesis of Alkynes 976
12.4 Ring-Closing Metathesis 978
12.5 Ring-Opening Metathesis and Ring-Opening Metathesis Polymerization 979
12.6 Practical Applications 983
References 986
Oligomerization and Polymerization 1001
13.1 Oligomerization 1001
Practical Applications 1006
Alkenes 1008
Alkynes 1013
Cyclooligomerization 1014
Practical Applications 1018
13.2 Polymerization 1021
Ziegler–Natta Catalysts 1038
The Phillips Catalyst 1041
Group IV Metallocene Catalysts 1042
Postmetallocene Catalysts 1047
Stereoregular Polymerization of Propylene 1058
Isospecific Polymerization 1059
Syndiospecific Polymerization 1064
Stereoregular Polymerization of Dienes 1065
Ethylene Polymers 1072
Polypropylene 1074
Polybutylenes 1075
Styrene Polymers 1076
Polydienes 1077
References 1078
Outlook 1111
14.1 Sustainable Hydrocarbon Chemistry for the Future 1111
14.2 Extraterrestrial Hydrocarbon Chemistry 1114
References 1115
Index 000
What People are Saying About This
“Highly recommended. Upper-division undergraduates through professionals.” (Choice, 1 March 2012)